Earth & Space
Rocks beneath volcanic hotspots can be surprisingly cool
Volcanic hotspots like Hawaii are usually thought to be fed by hot blobs inside the Earth. A new class of cold hotspots is identified by inferring the temperature from Earth’s CT-scan. Scientists need to figure out a new theory to explain its mysterious origin.
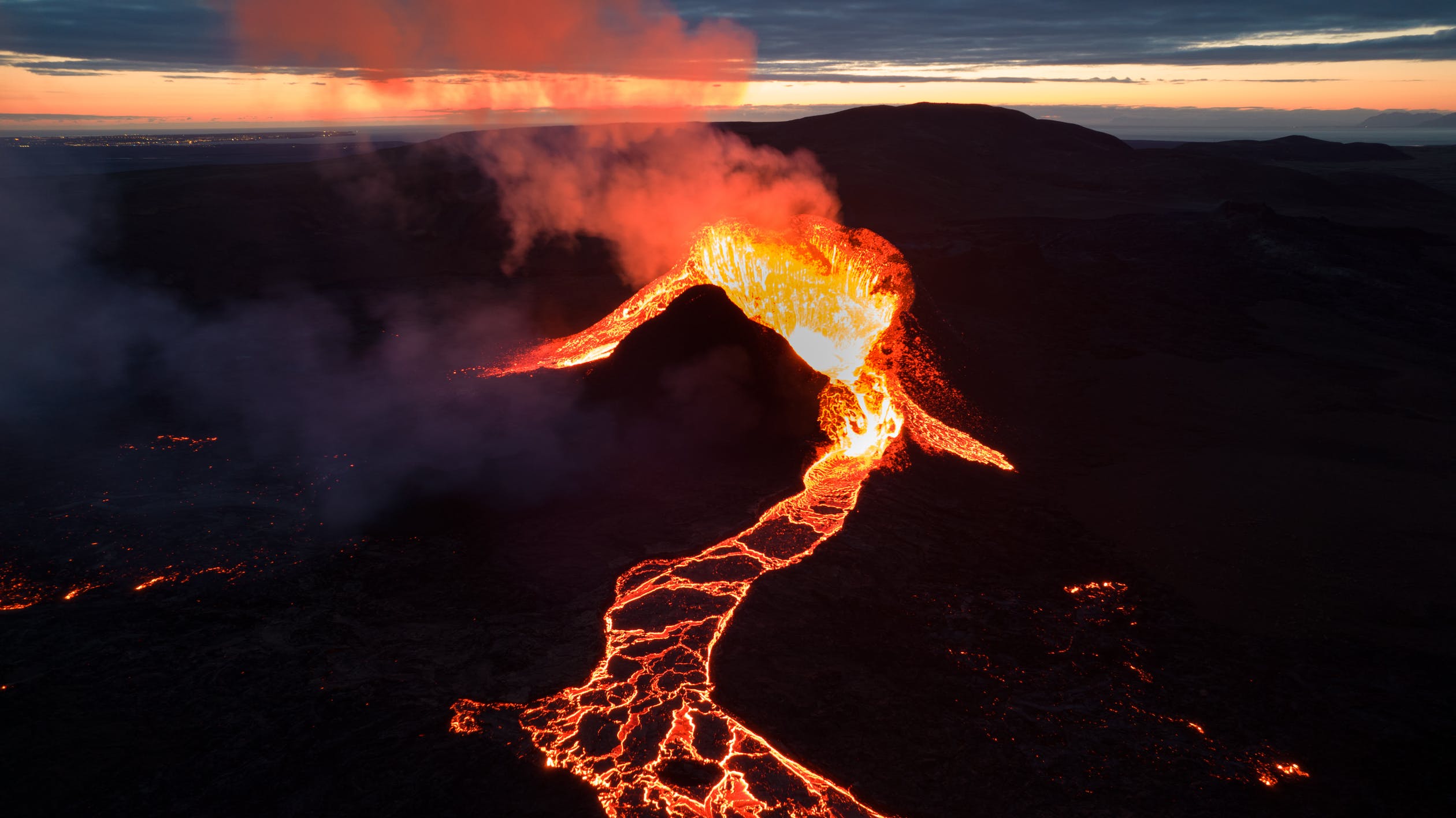
Volcanoes shape the surface of the Earth, by bringing molten rock from the deep interior to the surface and creating the crust. The majority of the Earth’s volcanism happens at plate boundaries, so called inter-plate volcanism, including the mid-ocean ridges (like the East Pacific Rise and Mid-Atlantic Ridge). Ridges are considered as the manifestation of the large-scale movement of rocks in the mantle, a 2900 km layer beneath the crust and above the core. As a result, sub-ridge mantle temperature represents the temperature of the ambient mantle.
On the other hand, intraplate-volcanism creates hotspots like Hawaii. The classical textbook explanation for volcanic hotspots is the mantle plume theory: the mantle is heated at the core-mantle boundary, and buoyant upwellings will then develop at the boundary. It is just like the heating the water in the kettle, except that mantle acts like viscous liquid over hundreds of millions of years. Such upwellings are faster than the large-scale flow in the mantle, and can reach the surface and produce seamounts and islands. One can see similar plumes in the heated syrup of honey.
According to the mantle plume theory, plumes need to be hot enough to be buoyant and rise fast. Therefore, they have to be at least 100~150 °C hotter than the ambient mantle (or ridges). It is extremely hard to directly measure the temperature in the mantle, since the deepest bole hole is still less than 13 km, while even the upper portion of the mantle extends to 660 km in depth. One can try to use the lava samples erupted at those hotspots to estimate the excess temperature over ridges, which is typically 100 ~ 300 °C. While this match the dynamic limit above, this type of thermometer suffers from the lack of samples, the limited coverage of global hotspots (about half), inconsistency among studies and the shallow source region (< 250 km depth). The temperatures of global hotspots thus remain inconclusive.
However, the mantle temperature is also hidden in earthquakes. Large earthquakes can trigger energetic sound waves in the rock, which travel very far away inside the Earth. These seismic sound waves change their speed based on the rock types, pressure, and temperature. In general, the higher temperature is the slower the seismic wave travels. Similar to the CT-scan of the human body, we can then use seismic waves to generate a 3-D image (tomography) of the Earth with seismic velocity. To get high resolution seismic tomography, we try to make full use of the seismic records available in our algorithm. Next, we take different physical properties and assembly of minerals in the rock into consideration, and we are able to infer the temperature of mantle beneath global hotspots and ridges simultaneously.
Surprisingly, we find that at least half of hotspots are either barely hot enough for plumes to rise fast (warm hotspots, excess temperature 50~136 °C), or as cold as mid-ocean ridges (cold hotspots, excess temperature ≤ 36 °C). Unlike the hot hotspots (such as Hawaii and Iceland), it is hard to explain the warm and especially cold hotspots identified (such as Ascension and Cameroon) using the classical mantle plume theory.
Instead of a single deep-rooted mantle plume beneath the hotspot, we believe secondary plumes branched from stronger plumes may feed warm hotspots which can be thinner, cooler, and may just originate from shallower mantle as well. We propose that if warm/cold hotspots are still plume-fed, the plume may be hot initially but trapped and cooled down by the ambient mantle. Alternatively, if they are not plume-fed, shallow heat transfer processes will then take the responsibility.
We also notice hot hotspots exhibit higher buoyancy flux (volume of seamounts), and higher helium-3/helium-4 ratio in the lava (an indication of ancient and possibly denser material). Both are consistent with our temperature estimates, since hotter plumes will be more buoyant and stronger, and they are able to entrain denser materials from the deep mantle.
To sum up, we identified a group of unexpected cold hotspots that do not fit the mantle plume theory. Modification to the plume model is required and shallow processes need to be involved. In the future, we would like to analyze each hotspot in more detail to better understand their temperatures, and perform computer simulations or experimental analogs to examine the cold hotspot models.
Original Article:
Bao, X., Lithgow-Bertelloni, C., Jackson, M. & Romanowicz, B. On the relative temperatures of Earth’s volcanic hotspots and mid-ocean ridges. Science 375, 57-61 (2022).Next read: A surprisingly geologically active Venus – evidence for recent volcanic and tectonic activity by Anna J. P. Gülcher
Edited by:
Isa Ozdemir , Senior Scientific Editor
We thought you might like
What the Earth’s ‘voice’ tells us about its underground architecture
May 25, 2021 in Earth & Space | 4 min read by Tim Stern , Simon LambMore from Earth & Space
Discovery of the first radiation belt beyond the Solar System
Jan 27, 2025 in Earth & Space | 3.5 min read by Juan Bautista Climent OliverOne million (paper) satellites
Jan 24, 2025 in Earth & Space | 3 min read by Ewan Wright , Andrew FalleVolcanic Ash: A Nutrient Boost for Reef-Building Corals
Sep 18, 2024 in Earth & Space | 4 min read by Frank Förster , Tom SheldrakeAmmonia Energy: A Call for Environmental Awareness
Aug 29, 2024 in Earth & Space | 3.5 min read by Matteo Bertagni , Robert Socolow , Amilcare PorporatoLikely increase in coral thermal tolerance at a Pacific archipelago
Dec 29, 2023 in Earth & Space | 3 min read by Liam LachsEditor's picks
Trending now
Popular topics